2.2. Rotary movements
One of the most studied natural nanomachines is certainly the one tasked with the synthesis of adenosine triphosphate (ATP), a chemical species which, as we have seen (Fig. 11), provides the energy for all muscle movements and therefore also presides over vital functions. This machine is 10 nm in size and is very complex (Fig. 13). Schematically, it is constituted by a cylindrical unit C, formed by long protein molecules wrapped in a helix, which crosses the cell membrane (wall), and by a unit γ fixed to C. When the concentration of hydrogen ions (H+) outside the membrane is higher than the concentration inside, a flow of hydrogen ions is generated through the C unit which thus begins to rotate as if it were a mill; the unit γ, which is integral to C, also rotates (Fig. 13a). The rotating γ, unit acts as a mechanical camshaft that successively deforms three sites in the α and β molecules that surround it, causing the transformation of ADP (adenosine diphosphate) into ATP in each of them (Fig. 13b).
In short, this natural molecular machine is a genuine rotary motor which, powered by a flow of hydrogen ions, produces mechanical work which in turn allows a low energy content substance (ADP) to be converted into a substance with a higher energy content (ATP). In organisms, therefore, cellular fuel (ATP) is totally regenerated from its own waste, thanks to a molecular machine. To get an idea of the amazing work done by these nanomachines, just think that one day an individual consumes about 70 kg of ATP, and that each ATP molecule is recycled on average 700 times.
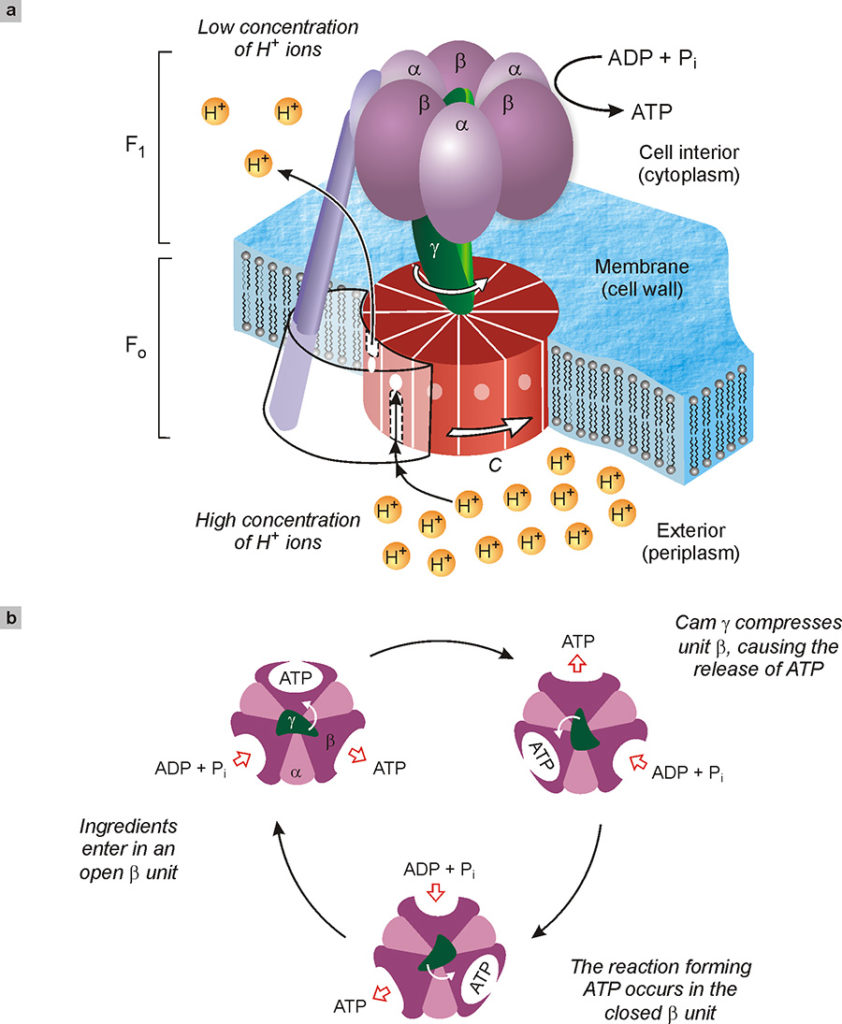
Fig. 13. Depiction of the enzyme ATP synthase tasked with the synthesis of adenosine triphosphate (ATP) starting from adenosine diphosphate, ADP, and inorganic phosphate (Pi). This enzyme, around 10 nm in size, consists of two rotating molecular motors, FO and F1, coupled together (a). In the normal functioning of the enzyme, a different concentration of hydrogen ions on both sides of the cell membrane causes a flow of the same ions through unit C. This flow rotates the unit C as if it were a mill. The γ cam, integral with C, presses successively on the catalytic units α and β of F1, causing the formation of ATP from ADP and phosphate ingredients. The top view of the enzyme (b) shows how the γ cam, by rotating, deforms in sequence the three sites where the synthesis of ATP takes place.
However, the operation of this nanomachine is even more complex and stunning. In fact, it combines two molecular motors, shown in Fig. 13a with F1 and FO, joined to the same cam γ. These two engines are both able to rotate, but in opposite directions. The FO, as mentioned above, exploits the flow of hydrogen ions to turn in one direction, while the engine F1, using the energy produced by the conversion of ATP into ADP (i.e. the reverse of the reaction mentioned above), rotates in the opposite direction. Since the two motors are connected in an integral manner, the direction of rotation is imposed by the strongest one. Thus, when the concentration of hydrogen ions inside the membrane is lower than outside (as it happens in bacteria due to a photosynthetic process), FO is the most powerful motor and F1 is forced to turn backwards. In chemical terms, this means that a current of hydrogen ions is “consumed” and the valuable ATP that supplies energy to the organism is produced. On the other hand, when hydrogen ions abound inside the membrane, the F1 motor prevails, forcing FO to turn backwards. In chemical terms, this means that the body can use the energy of ATP to pump the hydrogen ions where they are needed.
Motors made of natural proteins can be used to operate artificial mechanical devices. An example of this type is shown in Fig. 14 (Soong 2000). F1-ATP synthase units, taken from a bacterium, were chemically fixed on metal posts about 100 nm in diameter and 200 nm in height, manufactured by electron beam lithography. A nickel bar measuring 150 nm in diameter and about 1000 nm in length was attached to the γ cam of each F1 unit. When energy was supplied to the system in the form of ATP, the F1 nanomotors were found to rotate, dragging the metal bars in their motion. Since the latter are large enough to be observed with a normal optical microscope, and the metal supports are sufficiently distant from each other, this experiment permitted to “film” the functioning of single biomolecular motors.
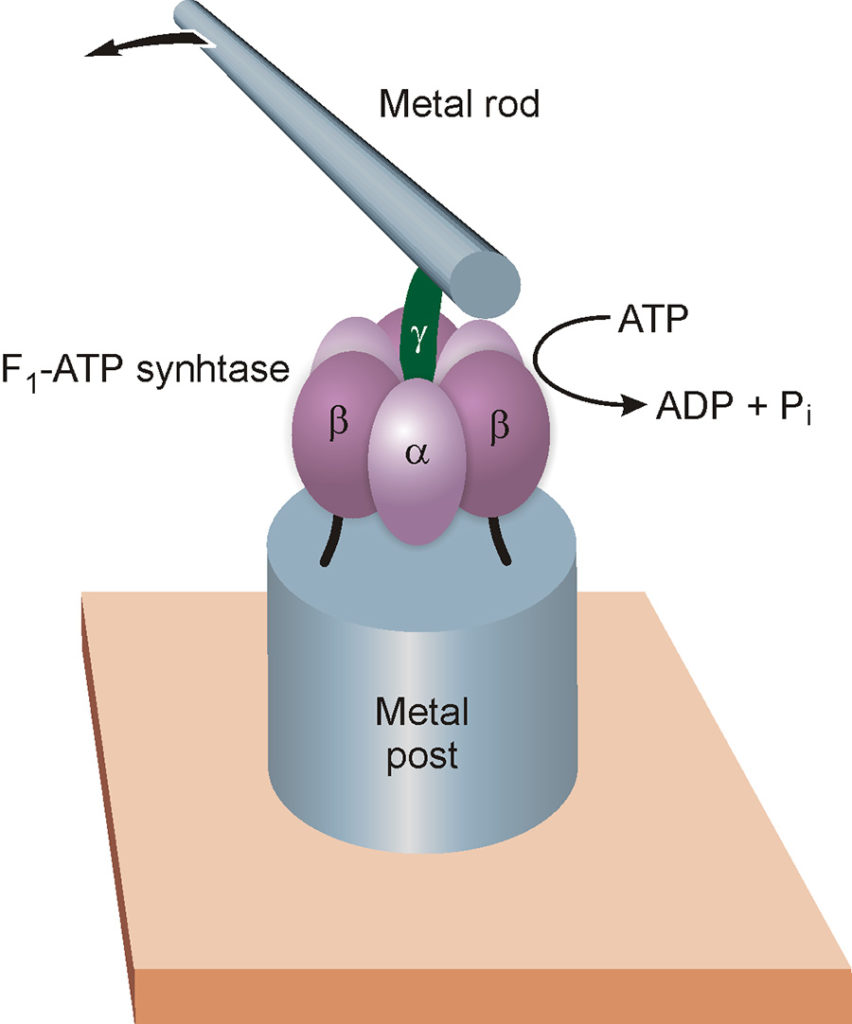
Fig. 14. The F1 part of the enzyme ATP synthase was used as an engine of an artificial nanodevice. The catalytic units α and β of the enzyme (see also Fig. 13) are fixed on a metal post with a diameter of about 100 nanometers (see text), while a metal bar about one micrometer long is tied to the γ cam. By administering ATP to the system, the molecular motor starts the cam, which in turn rotates the metal rod; the latter is sufficiently large to be observed under an optical microscope. It should be emphasized that the different parts of the illustration are not to scale: the metal bar is in fact about 100 times larger than the molecular motor to which it is connected.