4.6. Artificial muscles
In skeletal muscles a huge number of myosin molecular motors are organized according to a precise hierarchical structure (Fig. 10). Many myosin molecules form filaments, which, together with the actin filaments, constitute the sarcomere. Many sarcomeres, connected one after the other, give rise to myofibrils which, in turn, are associated in bundles, and so on up to the macroscopic muscle. The concerted action of billions of billions of myosin molecules, each of which develops a force of a few thousandths of a billionth of a Newton, is more than enough to raise, for example, the book you are reading. This observation contains an important message: with nanomachines one can do mechanical work in our world. What needs to be done is to insert a very large number of nanomachines in an organized structure, such that they can be stimulated simultaneously and their individual mechanical effects are added together. In practice, we need to build a system capable of amplifying movement from the nanometric to the macroscopic scale.
A clever strategy to achieve this is to combine molecular machines with polymer chemistry. Polymers are molecules of high weight – typically from a few hundred to a few thousand times that of the water molecule – obtained by combining smaller molecules (monomers), usually all of the same type or very similar to each other. Among the polymers present in nature there are proteins (or poly-peptides, obtained by combining amino acids, Fig. 9), nucleic acids such as DNA and RNA, and polysaccharides such as starch and cellulose. Synthetic polymers, more commonly known as plastics, are among the most important technological innovations of the last century and include materials we are constantly dealing with, such as polyethylene, polyvinyl chloride (PVC), polystyrene, polyesters (PET, polycarbonate) and polyamides (Nylon, Kevlar). From the repetition of many monomers (polymerization) it is possible to obtain linear macromolecules, consisting of a single chain of monomers, or branched macromolecules, in which different chains of monomers are connected to each other at various points.
In general terms we can think of using a molecular machine as a monomer for the construction of a polymer. For example, the research group led by Nicolas Giuseppone at the University of Strasbourg succeeded in obtaining a linear polymer (Fig. 31a) using artificial molecular muscles based on double rotaxanes (Fig. 23) as repetitive units. Experiments have shown that the polymer chains change their length by a few micrometers in response to the stimulation of their monomeric nanomachine components with acids and bases, demonstrating the amplification of molecular motion (Fig. 31a). More recently, applying the same strategy, Giuseppone and collaborators have constructed a branched polymer in which the distance between the branching points can be varied on command thanks to the molecular muscles (Fig. 31b). As shown in Fig. 31c, a fragment of this polymer shrinks when exposed to a basic solution, which causes the molecular muscles to pass from the expanded to the contracted form. After the subsequent treatment with an acid solution, the fragment regains its original size (Goujon 2017)1Goujon, A., Lang, T., Mariani, G., Moulin, E., Fuks, G., Raya, J., Buhler, E., Giuseppone, N. (2017) Bistable [c2] daisy chain rotaxanes as reversible muscle-like actuators in mechanically active gels, Journal of the American Chemical Society, 139: 14825-14828..
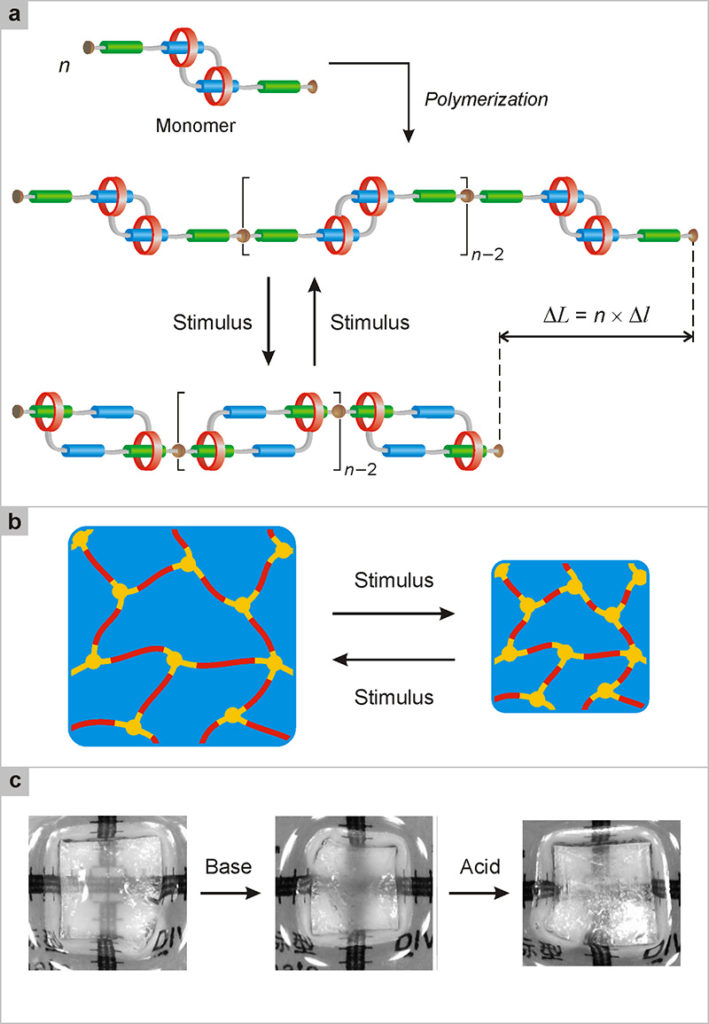
There are also systems of similar structure that use light as a source of energy for movement.
Equally fascinating and promising are the results obtained in branched polymers in which rotary nanomotors of the type shown in Fig. 26b and c have been inserted. As shown schematically in Fig. 32, by irradiating the material with ultraviolet light, the rotation of the molecular motors is activated and, consequently, the polymeric chains are twisted. This profound structural change, which affects the entire illuminated portion of the material, causes its contraction on a macroscopic scale (Li 2015)2Li, Q., Fuks, G., Moulin, E., Maaloum, M., Rawiso, M., Kulic, I., Foy, J. T., Giuseppone, N. (2015) Macroscopic contraction of a gel induced by the integrated motion of light-driven molecular motors, Nature Nanotechnology, 10: 161-165.. It is interesting to note that such a polymer is able to store part of the incident light energy in the form of chemical energy, associated with the entanglement of chains. The system represented in Fig. 32 has recently been modified by inserting in the ramifications, in addition to the rotary motors, also appropriate molecular dissipators that allow the unwinding of the tangled chains and the release of the accumulated energy. Plastics that work as mechanical actuators, based on molecular motors, are today a reality. The next step is to study possible practical applications in areas such as biomedical devices or robotics.
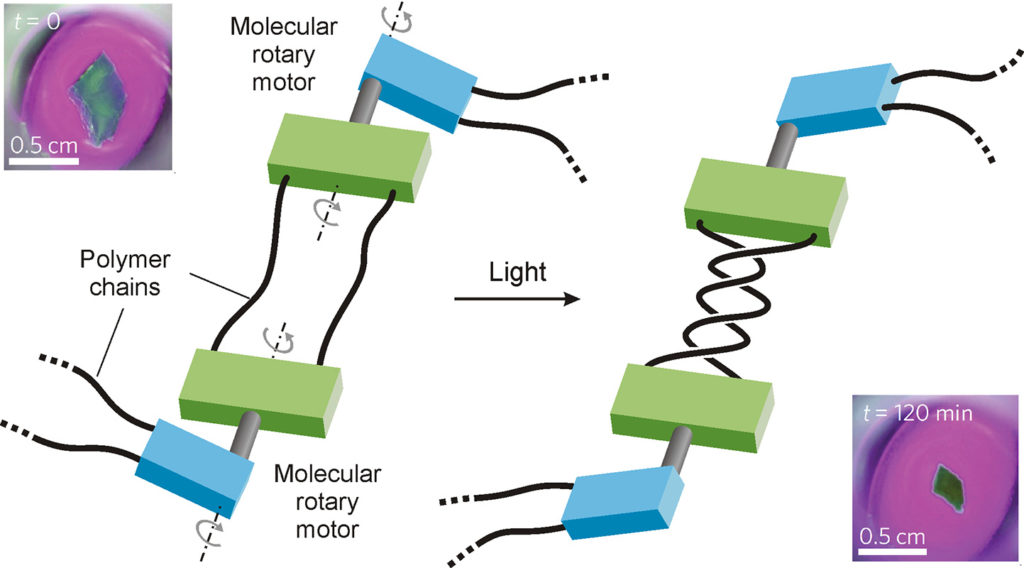